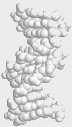

Teaching
Interests
I
am fortunate to have found a career that allows me to work hard at
things I love. As a graduate student teaching fellow, I first discovered
that I was equally stimulated by the intellectual challenges of research
and of explication. I am therefore happy to be able to combine these
activities at institutions that value quality teaching as well as
scholarship. I enjoy teaching broadly. In addition to several courses
for Biology majors at both the introductory (Introduction to Genetics
and Development, Introduction to Experimental Biology) and advanced
(Genetics, Cell Biology, Developmental Biology) levels, I have designed
and implemented several classes for non-biology majors: At Home in
the Universe, a Scientific World View; Remaking Eden. I feel that
my exposure to a wide spectrum of students with diverse interests
and backgrounds has significantly enriched my pedagogy.
I
find that the primary challenge in teaching science, regardless of
audience level, is to pique student interest in a particular phenomenon
to such a point that learning about the intricacies underlying the
phenomenon will be fun for them. I always try to follow Einstein's
axiom, "Science should be made as simple as possible, but not
simpler." Given the complex nature of biological processes, it
is important to grab student interest in a topic early on, so that
their curiosity will see them through difficult material. I try to
accomplish this through several pedagogical practices.
First,
I try to share my own wonderment about the natural world and the way
that evolution by natural selection has fashioned life's processes,
and in this way to forge student enthusiasm for learning.
Second,
I believe it is essential to examine the multiple facets of a topic
at hand. For example, in discussions of genetic recombination, I make
sure that students learn about the evolutionary implications of gene
mixing in addition to the consequences of this process in inheritance.
This is followed by a treatment of the macromolecular machinery involved
in recombination, so that students gain an appreciation for the way
that this process may have evolved from earlier DNA repair mechanisms.
In short, by making the connections between various sub disciplines
explicit, I emphasize the centrality and relevance of a particular
topic, casting the net for student interest as widely as possible.
Third,
I am a tireless advocate for visualization as a learning tool for
students. For example, molecular graphics are especially important
in teaching current biology, because so much about the function of
biological molecules can be conveyed with molecular models. As Oliver Morton has written, "Biology is a way of structuring matter at a molecular scale by slotting each atom into its needful place" - The Economist, 2019. I have
created, with student collaboration, a WWW site for the study of macromolecular structure, The Online Macromolecular Museum: www.callutheran.edu/BioDev/omm/gallery.htm. The OMM's exhibits are interactive tutorials on individual molecules
in which hyper textual explanations of key biochemical features are
linked to illustrative renderings of the molecule at hand. The OMM
has proved to be a valuable resource for my students and is in widespread
use. I've received positive feedback on the OMM from colleagues and
students worldwide, and the OMM has won widespread acclaim. Another
example is the use of confocal/deconvolution microscopy in our biology
curriculum, allowing our students to engage in some of the eye-popping
learning experiences that modern microscopy affords.
Fourth,
I use information technology (IT) intensively and in varied contexts
in my classes. I think that IT significantly enhances communication
and collaboration between my students and me (and among students)
and empowers student learning in ways not possible with conventional
means. I have recently embarked on a large-scale reform of my lecture
classes by implementing and studying the impact of the transformation
of these from a traditional model involving classroom lectures and
textbook readings assigned as homework to an inverted (“flipped”)
design, CLIC (see figure, below). In the CLIC model, students view
engaging cinematic lectures outside of class, enabling the transformation
of classrooms into arenas of inquiry and active learning (CLIC = Cinematic
Lectures & Inverted Classes).
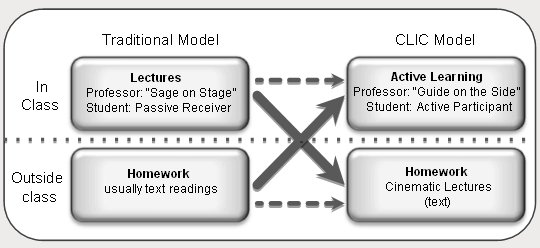
Inversion
of lecture courses from a traditional
to an inverted format. In the CLIC model (CLIC = Cinematic
Lectures & Inverted Classes), students view online, cinematic
lectures that incorporate multiple media outside of class
(with optional text consultation) and participate in collaborative,
inquiry- and problem-based activities in class. This transforms
the standard roles of both student and professor, the latter
of which is a long-advocated switch from “Sage on Stage”
to “Guide on the Side”
Finally,
I am firmly committed to involving my students as teachers and not
just learners. As Seneca wisely wrote, "we learn while we teach."
When brief lectures are required, I employ Socratic questioning
frequently, having students put in their own words their understanding
of a key point, and placing them in the position of explaining a concept
to their peers. This not only gives me useful feedback on the class'
progress, but also teaches students to "speak Biology,"
improving their oral communication skills. In seminars, I become a
facilitator and encourage my students to take over much of the discussion.
Many of my courses involve intensive projects in which students make
formal presentations that are open to the public. This approach reinforces
a student's sense of responsibility for his/her own education, empowers
students to become young educators, and invariably increases their
interest levels.
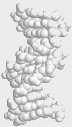

Philosophy
of Science Education in Liberal Arts Setting
What is liberal learning? Perhaps a roomful of academics might debate
the answer to this question for sometime, but I think most would agree
that one defining feature of a liberally educated student is, in the
words of Eva Brann : "
[that they have learned] how they
came by the opinions they bring along, so that they may be able to
choose whether to hold on to them or to change them." Or, as
Malcolm Forbes once quipped, "Education's purpose is to replace
an empty mind with an open one."
I
hold that an essential component of opening minds is their exposure
to diverse worldviews. Sadly, only a small percentage of American
college graduates today have the opportunity to learn, in depth, about
the worldview that sums up our current understanding of the natural
world, including ourselves, after ~ 350 years of post Enlightenment
science. So, in my view, an imperative for science education in a
liberal arts setting is to provide students a broad scientific erudition.
At Kenyon College, I developed and directed a year long, interdisciplinary
science class titled "From Cosmos to Consciousness - A Scientific
Worldview" to promote such knowledge. At CLU, the course has
morphed into a year-long, team-taught class for honors students, "At
Home in the Universe."
Biology
is a very important component of such a course because the philosophical
implications of modern Darwinism are under appreciated in many parts
of our global society, including the United States. The philosopher
Daniel Dennett describes the effects of these implications : "[Darwinism
is]
a universal acid; it eats through just about every traditional
concept and leaves in its wake a revolutionized world view."
The breakthroughs in genome research over the past decade have provided
us direct readings of the documents of evolutionary history. What
these documents (plus a vast empirical fundament of prior knowledge
obtained in various subdisciplines of Biology) tell us is that life
on earth does not bear evidence for intelligent design, but rather
that the design we see in living creatures arises from the random
processes of mutation, genetic recombination and genetic drift coupled
with the very NON-random force of natural selection. These considerations
of the "blind watchmaker" model of biological evolution
often, and delightfully, lead to thought provoking discussions with
open-minded students who might initially find them counterintuitive.
A valuable outcome of these discussions is the realization that a
scientific worldview provides a very soulful, awe-inspiring perspective.
Contemplation of the indisputable and non-metaphorical kinship of
all life on earth can be a very edifying experience indeed. It is
my contention that a scientific worldview can be an important component
of a set of ethics that is evidence-based, one that is infused with
a deep respect for humanity and its companions on our fragile planet,
and one that informs a rational approach to solving some of our most
vexing problems.
Liberal
education goes further than simply immersing students in a variety
of worldviews, however. John Seely Brown and Paul Dugid , in arguing
against large scale distance education and for maintaining the physical
continuity of residential colleges and universities, posit that high
quality education involves the enculturation of students as young
scholars into learning communities (something that distance learning
simply is not good at). This enculturation, at its best, involves
students deeply in creative processes, irrespective of their chosen
discipline or future career goals. In the words of the Boyer Commission
on Undergraduate Education, we need to transform our educational programs
from "
a culture of receivers into a culture of inquirers."
I believe it is to this culture of inquiry, a true hallmark of quality
liberal education, that we wish to invite our students. I enthusiastically
contribute to this culture by conducting collaborative research with
undergraduates, an activity that is some of the most satisfying teaching
I do. The opportunities to engage small groups of students directly
in the scientific enterprise and to share with them the excitement
of creating new knowledge are continual sources of joy for me. These
activities are the backbone of an experiential curriculum, for there
is no better way to learn science than by doing science. I am proud
of the accomplishments of my numerous research students, and am grateful
to them for allowing me to serve as their mentor.

Research
Interests
Discovering
the mechanisms of developmental processes in Drosophila melanogaster
has provided key insights into animal development in general, and
human development in particular. For this reason, this model organism
continues to play an important role in biomedical research. We are
investigating aspects of head development in D. melanogaster that
are relevant to the mechanisms by which tissues are partitioned into
distinct developmental fields.
The compound eye of Drosophila melanogaster consists of about 800
ommatidia in a polar arrangement around the dorsoventral (D-V) midline.
Each ommatidium consists of eight photoreceptor cells arranged in
a trapezoidal fashion with two mirror-symmetric forms, a dorsal form
above the D-V midline, and a ventral form below. When differentiation
of the ommatidia begins within the epithelium of the third instar
larval eye-antennal imaginal disc, each ommatidium is a bilaterally
symmetrical cluster of photoreceptor precursors polarized in the anteroposterior
axis. These precursors become polarized on the D-V axis by proto-ommatidium
rotation. The establishment of polarity along the D-V axis requires
the JAK-STAT signaling pathway, which is activated by a ligand encoded
by unpaired (upd) (Zeidler, et al., 1999). Hopscotch (hop) is the
Drosophila ortholog of mammalian JAK (Janus Kinase), a Protein Tyrosine
Kinase (PTK).
Several reports suggest that JAK-STAT signaling is important in establishing
the nascent ommatidial field. First, over expression of hop in developing
head tissue yields profound defects, including the production of extra
eyes on dorsal aspects of the adult head (Harrison, et al., 1995).
Second, Notch induced expression of eyegone, a Pax transcription factor,
is required to establish and organize the prospective eye field. Eyegone,
in turn, induces expression of upd at the D-V midline, activating
the JAK-STAT pathway over long distances in the developing eye (Chao,
et al., 2004). Third, regulatory mutations of upd yield a small eye
phenotype (Zeidler, et al, 1999).
The mutant defects resulting from the over expression of the hop-encoded
PTK in the developing head are similar to the ectopic compound eye
duplications caused by the extra eye (ee) mutation (Marcey and Stark,
1985). The ee mutation is likely caused by a P-element insertion into
a 5’ exon of the Cpr gene, which encodes a P450 oxidoreductase.
This insertion places the P-element in a reverse orientation with
respect to transcription polarity of the Cpr gene, which results in
the presence of anti-sense P-element RNA within the Cpr transcript.
The ee mutation displays several interesting genetic features. First,
the mutation is incompletely penetrant (not all flies homozygous for
the mutation display a mutant phenotype). The mutation is variably
expressive in that phenotypes can include duplicated antennae, bristles,
or eyes. Finally, ee is conditionally dominant, meaning that heterozygotes
can display the mutant phenotype. ee has been shown to be dependent
upon ee enhancers, which are P-elements at various genomic positions.
Based on this and other data, we have developed a model to explain
the exotic genetic behavior of ee that posits a down regulation of
a negative regulator of the JAK-STAT pathway, Su(var)2-10, as a consequence
of the P-element insertion into Cpr and subsequent RNAi-induced heterochromatization
of the Su(var)2-10 genomic region due to a nearby P-element insertion.
Several studies support this model. First, a component of the ee mutation
behaves genetically as an allele of Su(var)2-10, acting as a suppressor
of position effect variegation (see Betz, et al., 2001), thus strengthening
the hypothesis that Su(var)2-10 is down regulated in ee. Second, mutant
alleles of Su(var)2-10 act as ee alleles when combined with the ee
chromosome, a corollary to the first result. Third, it has been shown
that mutants which decrease heterochromatization, pleiohomeotic (pho)
and brahma (brm), exhibit a significant suppression of ee penetrance,
indicating that the level of heterochromatization may influence ee
penetrance, putatively through effects on Su(var)2-10 expression.
Further
tests of our model using genetic, molecular, and cell biological approaches
are underway.
Cryptic genetic variation (CGV) may be an important component of adaptive, deleterious, or neutral variation that is contingent upon environmental or genetic circumstances to be expressed. CGV therefore qualifies as a “hidden substrate” of evolution (Paaby and Rockman, 2014). Inducible epigenetic variation (IGV) is a subset of CGV that may be revealed upon changes in the packaging state of genes or their regulatory components as opposed to DNA sequence variation that is contingently expressed.
We are conducting screens for novel sources of inducible genetic variation (IGV) in natural populations of Drosophila melanogaster. IGV may be an underappreciated component in the spectrum of natural variation upon which natural selection can act. The screen is based on the model of Figure 2. The proposed epigenetic model predicts that new mutant phenotypes may be uncovered in crosses between ee lines and flies from various natural populations that harbor P-elements at different genomic locations. Such novel mutants are predicted to be incompletely penetrant and variably expressed, caused by epigenetic silencing via RNAi-induced heterochromatization of genes residing at genomic positions near P-elements. In an initial study described here, we conducted a screen for such mutations by scoring progeny of crosses of separate, wild-derived lines to ee.
In one such cross, a new mutation, crybaby (cby), was recovered (Figure 4). Cby exhibits the properties expected of an epigenetically-triggered mutation. Our results to date suggest that variation in natural populations may include cryptic, epigenetic sources linked to transposable elements, which are revealed under particular genetic contingencies. Screens of multiple wild strains for additional examples of cryptic phenotypes are ongoing, as are genetic and molecular characterization of the cby and ee phenotypes.
The recovery of a mutation that displays signs of an epigenetic nature in 1/16 crosses of extra eye to wild-type stocks comports with our prediction that such mutations would be revealed in our screen. We posit that such mutations are caused by epigenetic suppression of genomic regions near P-element inserts that is rooted in RNAi mediated transcriptional gene silencing caused by the antisense P-element in the Cpr gene in extra eye lines. Future studies will be aimed at screening a large number of lines derived from additional geographic locations, and studying the crybaby mutation in detail at both the genetic and molecular levels. We will determine the map position of crybaby and predict that a major genetic component is coincident with the extra eye P-element in Cpr. Our hypothesis concerning hidden epigenetic variation in natural populations, if confirmed by additional work, has significance for gene regulation and evolutionary biology as this type of variation may provide new “epialleles” that are manifested in the presence of an appropriate antisense transposable element. Such “epialleles” would provide natural selection a collateral source of variation upon which to act, in addition to DNA sequence changes in coding or regulatory regions of eukaryotic genes.
References
Chao,
J.-L., et al. Localized Notch signal acts through eyg and
upd to promote global growth in the Drosophila eye.
Development. 2004; 131: 3839-3847
Harrison,
D.A., et al. Activation of a Drosophila Janus kinase (JAK)
causes hematopoietic neoplasia and developmental defects. EMBO J.
1995; 14(12): 2857-65.
Marcey,
D.J. Stark, W.S. The Morphology, Physiology, and Neural Projections
of Supernumerary Compound Eyes in Drosophila melanogaster.
Developmental Biology. 1985; 107: 180-197.
Edwards,
K., T. Davis, D. Marcey, J. Kurihara, D. Yamamoto. 2001. Comparative
Analysis of the Band 4.1/ezrin-related Protein Tyrosine Phosphatase
Pez from Two Drosophila Species: Implication for Structure and Function.
Gene 275: 195-205.
Shuai,
K. Liu, B. Regulation of JAK-STAT signaling in the immune system.
Nat. Rev. Immunol. 2003; 3(11): 900-11.
Zeidler,
M. P., Perrimon, N. and Strutt, D. I. Polarity determination in the
Drosophila eye: a novel role for Unpaired and JAK/STAT signaling.
Genes Dev. 1999; 13: 1342-1353.
|